(MENAFN- Asia Times) This is the second of a three-part series.Click here to read part one, which relates how early experimentation in cold fusion was largely abandoned due to disappointing results when researchers attempted to replicate findings – but the second wave of research is now showing promising results.
In my view Japan – without question the world leader today when it comes to experimental research in this field – has produced the most compelling demonstrations of the existence and reproducibility of cold fusion.
Japan owes its leading position in large measure to consistent institutional and industrial support and a systematic, step-by-step approach emphasizing the development of advanced materials for cold fusion devices. Cold fusion research lies at the intersection of nuclear physics and materials science, and Japan's successes in cold fusion would be impossible without a strong industrial base in the fields of nanomaterials and nanotechnology.
The Japanese effort has also profited from the leadership provided by Akito Takahashi of Osaka University, known for his work on hot fusion and other areas of nuclear science, who has been actively involved in cold fusion since its earliest days.
A watershed was reached two years ago with the completion of a multi-institutional project sponsored by the New Energy and Industrial Technology Development Organization (NEDO), one of the largest public funding agencies in Japan, working under the Ministry of Economy, Trade and Industry. The project involved a collaboration among Kyushu University, Tohoku University, Nagoya University, Nissan Motor Co and Technova (a technology firm in which Toyota Motor Corp is a principal shareholder).
The series of 16 collaborative experiments aimed to clarify the nature of 'the anomalous heat generation phenomena' in hydrogen-saturated metals, and to reproduce these phenomena in a consistent manner. For this purpose the collaborative effort focused on a technology that Japanese scientists have brought to a high level of maturity: gas loading of specially-prepared 'nano-structured materials."
It is worth describing this method in a bit of detail so that the reader can get a better idea of how cold fusion energy-generation technology might look in the future. Gas loading is an alternative approach to that originally taken by Fleischmann and Pons. The sample material is placed in a closed chamber, which is then filled with hydrogen (or deuterium) gas under pressure, causing a portion of the hydrogen to be absorbed into the sample. Given the right choice of materials gas loading can achieve a high density of absorbed hydrogen nuclei in the sample.
In the relevant experiments, heating elements were installed in the chamber so that experiments could be carried out both at room temperature and at temperatures in the 100-450°C range.
As testified by nearly three decades of experience, the principal key to success lies in the choice and preparation of the sample material. What counts most is the detailed structure on the scale of microns down to nanometers – millionths of a millimeter.
Whether or not the cold fusion effects occur, and how large they will be, depends on the precise geometry of the sample's crystal structure, on the type and density of defects and impurities, their positions in the crystal lattice, on surface characteristics, etc.
In ordinary industrially produced metals the microstructure and nanostructure can differ very greatly from one batch to another. 'Palladium is not palladium!' No two palladium bars are alike in their microstructure; each one retains in its structure a memory of its entire past.
This circumstance – together with failure to attain a sufficiently high density of hydrogen in the sample – explains in great part why past attempts to reproduce the results of cold fusion experiments have so often ended in failure.
Accordingly, Japanese scientists invest great effort into 'engineering' specialized materials for cold fusion, using production methods that make it possible to control the nanostructure of the sample to a high degree.
The NEDO program experiments employed metal composite powders synthesized from various combinations of the elements copper, nickel and palladium, in the form of nanoparticles embedded in larger (micrometer-sized) particles of zirconium and silicon oxide.
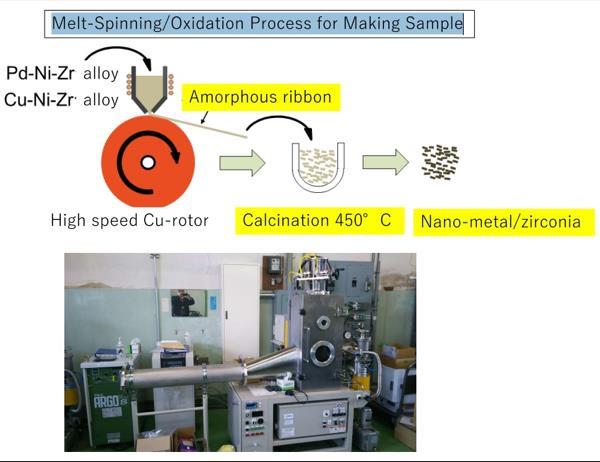
Illustration of materials preparation for cold fusion experiments, courtesy of Yasuhiro IwamuraAs a demonstration of reproducibility, samples of palladium-nickel-zirconium oxide powder, taken from a single batch of production, were employed in independent parallel test runs at laboratories at Kobe University and Tohoku University. Both laboratories observed sustained excess heat over a period of more than 10 days. The data were qualitatively and quantitatively similar between the two laboratories.
Other collaborative experiments verified the continuous generation of excess heat over periods ranging up to a month. All 11 experiments using the specially-prepared palladium-nickel-zirconium and copper-nickel-zirconium samples demonstrated net heat production. The total amounts of energy released were larger per atom than in any known chemical reaction, sometimes by a factor of several hundred.
In the course of these experiments, the Japanese researchers verified the existence of other phenomena frequently reported from cold fusion experiments, such as occasional releases of energy in the form of sharp bursts.
Important for future commercial applications was the confirmation that comparable – if slightly lower – amounts of energy could be obtained using ordinary hydrogen rather than deuterium, which is much more expensive to produce.
The results of the NEDO project come as no surprise to anyone who has followed the Japanese cold fusion effort. This effort has produced a plethora of similar or quantitatively even better results over recent years.
Another chapter of cold fusion research in Japan concerns the perspectives for utilizing cold fusion-related technology to neutralize high-level radioactive waste from nuclear power plants, including Fukushima. The basic idea was suggested by the frequent observations of the transmutation of elements in cold fusion experiments.
For more than a decade Mitsubishi Heavy Industries supported research effects in this direction, and extremely promising results were published. More recently the MHI group, led by Yasuhiro Iwamura, has been transferred to a new Condensed Matter Nuclear Research Center at Tohoku University.
Google comes on board While the Japanese quietly continue their step-by-step progress toward commercial applications, the most sensational news about cold fusion has come from America.
On May 27 of this year the world's most prestigious scientific journal, Nature, published a detailed research article on cold fusion, co-authored by scientists from the Massachusetts Institute of Technology (MIT), the University of Maryland, Lawrence Berkeley National Laboratories, the University of British Columbia, the Canadian Institute of Advanced Research – and Google Inc.
It would have been sensational enough for Nature to publish any research at all concerning this supposedly discredited area, but the presence of a Google representative on the list of authors guaranteed a second surprise. And a signal, too. The collaborative research reported in the article was sponsored by Google with some collateral support from federally-funded scientific institutions of the US and Canada.
Entitled 'Revisiting the cold case of cold fusion," the Nature article describes the preliminary results of a collaborative effort designed to take an independent, fresh look at cold fusion.
The Google-sponsored program was launched in 2015. It assembled a new network of research teams, which embarked on designing and carrying out experiments to test the validity of key claims made by cold fusion experimenters. Experienced old veterans of cold fusion research were deliberately not included in the teams.
The Nature article emphasizes the Google teams' efforts to resolve difficult technical issues that have plagued cold fusion experiments from the beginning, noting that 'a few years, not just a few months, were going to be necessary to construct the requisite apparatus and conduct statistically significant numbers of experiments."
So what are the results? So far nothing, according to the authors – no sign so far of the cold fusion phenomena selected for study. Some readers might conclude: 'Aha! One more proof that cold fusion does not exist!' But this is obviously not the conclusion the authors themselves draw.
In fact, as I learned at the ICCF-22 conference, the Google-sponsored effort remains ongoing and is actually being expanded. So why publish such an article now? Clearly the purpose lies not in the experimental results per se – which are just preliminary – but rather to break the taboo against cold fusion research in the scientific community. And to draw more people into the effort.
The article in Nature concludes with an unusual 'call to action," in which the authors state, in part:
The underlying motivation of our effort is that our society is in urgent need of a clean energy breakthrough. Finding breakthroughs requires risk-taking, and we contend that revisiting cold fusion is a risk worth taking. We hope our journey will inspire others to produce and contribute data in this intriguing parameter space.
This is not an all-or-nothing endeavor. Even if we do not find a transformative energy source … the search for a reference experiment for cold fusion remains a worthy pursuit because the quest to understand and control unusual states of matter is both interesting and important.
Feeling the elephant That brings us to a few words about the theoretical side of cold fusion research. At present, there is no single, experimentally-proven theory explaining the phenomena observed in cold fusion experiments. Instead, we have a variety of interesting hypotheses, many of which contradict each other.
I am reminded of the ancient proverb of 'blind men feeling the elephant." Feeling different parts of the animal with their hands, each of the blind men comes to a different conclusion. Without a plausible theory to guide them, cold fusion experimentalists are also groping in the dark.
In this context, one of the highlights of the ICCF-22 conference was a series of presentations by Peter Hagelstein and his collaborators at MIT, where new activities in the domain of cold fusion are being planned. Hagenstein's theory has the advantage of predicting important and testable physical effects that are not directly connected with cold fusion per se.
The central issue of any cold fusion theory is to understand how the behavior of an atomic nucleus changes when the nucleus is located in the dense and highly structured environment of a crystal.
Up until recently, nuclear physics has nearly entirely ignored the possible influence of such an environment on what we might term the inner life of a nucleus. Nuclear physics and solid-state physics were in this sense regarded as completely separated disciplines.
According to Hagelstein, however, modern quantum theory provides for the existence of a coupling between a nucleus and vibrations of the crystal lattice in which it is embedded – vibrational waves known as phonons.
Among other things, nuclei can transfer large amounts of energy to phonons, which would finally appear as heat rather than high-energy radiation. Applied to nuclei which have just been formed by a fusion reaction, this could explain the absence of large amounts of radiation in cold fusion experiments.
Also, the ability of nearby nuclei to interact with each other via phonons might provide a mechanism for nuclear reactions such as fusion to occur at vastly higher rates in a crystalline environment. All of this points to a possible explanation of cold fusion.
Independently from that, however, Hagelstein's theory predicts the possibility of a transfer of energy from one nucleus to another across a considerable distance within a crystal. Subsequently, the MIT group was able to obtain strong experimental evidence for exactly this phenomenon.
The newly-discovered form of 'nuclear excitation transfer' in crystals could have significant technological applications of its own. Here we have one of many examples of potential spin-offs of cold fusion research.
To read part one of this series, clickhere . Next, part three: Asia and the commercial future of cold fusion – it's not just Japan; India and China may become players
MENAFN1611201901590000ID1099282025