(MENAFN- Asia Times) In the first part of this series, we saw that electromagnetic processes in plasmas – electrically conducting gases – could, over trillions of years, produce the giant filaments that we see today as the largest structures in the universe.
This happened without a Big Bang, without dark energy or dark matter, based on processes that we observe here on Earth in the laboratory and in space at all scales.
But the process of filamentary growth only produced huge filaments of current and plasma. How did these vast filaments of plasma in turn produce the glowing hierarchy of planets, stars, galaxies, clusters and superclusters of galaxies that we see today?
In this second part of the series, we see that different processes, still based on well-known physics, produced today's hierarchy of cosmic structure.
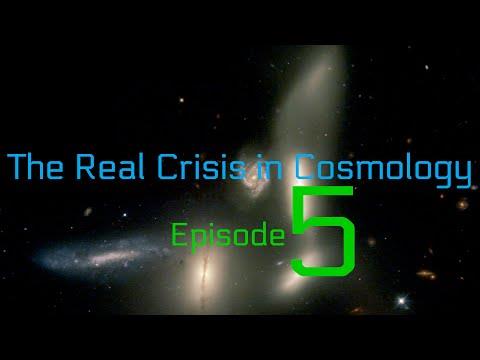
Readers are alerted that this installment in parts is more technical than usual. For help in understanding the discussion below, have a look at Lerner's video presentations, this one and another that's embedded farther down. – Jonathan Tennenbaum, Asia Times science editor The Universe as electric generator The process that became important when the vast current filaments formed was gravitational contraction. For fundamental reasons, as plasma pioneer Hannes Alfvén pointed out, plasma phenomena have certain characteristic velocities at all scales. Thus huge filaments resemble tiny filaments, just bigger. But the force of gravity gets larger on larger
scales, so that on immense scales of billions of lightyears gravitational forces became comparable to magnetic forces.
This follows directly from the laws of gravitation, known back to Newton's day. Gravitational forces, negligible for filaments light years or even millions of light years across, became dominant when the filaments reached billion-light-year size.
So, gravity started to form blobs of plasma, contracting along the axis of the largest filaments. The centrifugal forces of the spinning filaments resisted gravity toward the axis, but not along it, so disk-shaped blobs formed. But such disks set up a new interaction with the magnetic field.
As Michael Faraday discovered two centuries ago, a conducting disk rotating through a magnetic field produces electrical currents that move from the circumference of the disk toward its center. (This Faraday disk generator was the first way invented to turn energy of motion into electrical currents.)
These disks of plasma, spinning through the magnetic field of the huge filaments, generated a new set of currents flowing towards the axis and a new set of filaments on a smaller scale.
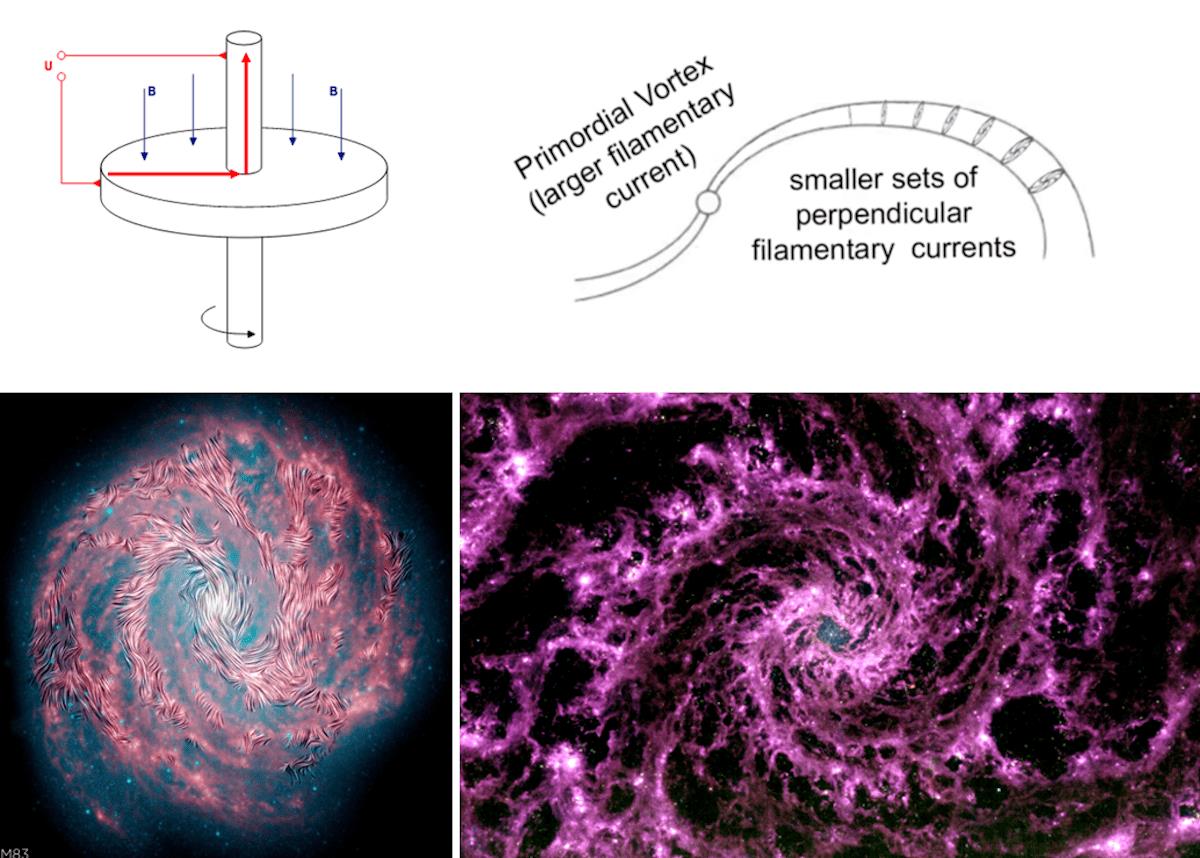
As the disks of plasma formed by gravity rotated in the magnetic fields of the filaments they generated currents that flowed inward and then out along the axis (upper left), leading to the formation of a smaller set of inward-flowing filaments (upper right). We see this hierarchy of electric currents and fields today in galaxies like M83 (lower left, where the directions of fields and currents are indicated by the superimposed lines), creating the structures of glowing stars seen in galaxy NGC 628, imaged in the infrared (lower right). Images: LPPFusion / NASA.
These filamentary currents were extremely important, as they transferred large amounts of angular momentum from the inside of the disk to the surrounding plasma. Isolated objects spin faster as they contract, like a skater drawing in her arms, because angular momentum is conserved.
Gravitational fields can't transfer angular momentum, so gravitation alone can't cause object to contract very much – they quickly spin fast enough to counteract gravity. But magnetic fields can convey angular momentum and the inward-flowing filaments act like giant baseball bats, accelerating the outer plasma they hit and, by reaction, slowing down the inner plasma, allowing objects to contract substantially, losing their spin.
When the filamentary currents converge in the center of the collapsing object, they bend at right angles to exit along the axis. At this point, another instability process takes over and the filaments start to kink together, winding themselves up into a dense ball of plasma called a plasmoid.
The interacting electromagnetic process in the plasmoid generates huge electrical fields, accelerating beams of atomic nuclei (protons) in one direction and electrons in the other along the axis. These spinning beams also carry large amounts of angular momentum, allowing the centers of the objects to contract further.
MENAFN10122023000159011032ID1107567444
Legal Disclaimer:
MENAFN provides the information “as is” without warranty of any kind. We do not accept any responsibility or liability for the accuracy, content, images, videos, licenses, completeness, legality, or reliability of the information contained in this article. If you have any complaints or copyright issues related to this article, kindly contact the provider above.